Deep dive into four technological areas
6.1 Reducing enteric methane emissions via methane inhibitors
The use of methane inhibitor feed additives has the potential to lower methane emissions from livestock and is thus one tool to slow the progression of climate change in the short term. Long-term emissions reductions will necessitate less food loss and waste and a protein transition: both shifting toward more sustainably produced livestock products, and also dietary shifts to lower meat consumption – where this is very high – and greater consumption of both plant and alternative proteins, which have less environmental impacts.
Global methane emissions need to be greatly reduced to limit warming to less than 1.5°C or 2°C, but unlike carbon dioxide do not need to fall to zero by 2050 (IPCC 2022). Climate Action Tracker has set the 1.5°C-aligned target for agricultural production emissions as a 39 percent absolute reduction by 2050 relative to 2017 (Climate Action Tracker 2023). They note that, as global population and food demand are projected to continue growing through at least the year 2050, the emissions intensity of agricultural production per calorie of food produced will need to fall even faster than this 39 percent absolute target.1 Rapidly reducing the emissions intensity of livestock is therefore critical by 2030.
One way to reduce methane emissions from the digestive process of ruminant animals, such as cattle, sheep, and goats, is through the development of feed additives that lower emissions by interfering in the processes that generate methane. Feed additives that lower rumen methane production fit two classifications:
- Rumen modifiers that modify the rumen microbial ecosystem with some resulting in >30 percent methane-reducing efficacy.
- Compounds that inhibit methane-producing enzymes reduce methane production by 30–95 percent, depending on type, dose, and duration of use (Cornell CALS 2024).
In the last five years, there has been significant progress in the development of methane inhibitors (GRA 2021), including 3-nitrooxypropanol (3-NOP, trade name Bovaer®) made by DSM (dsm-firmenich), Agolin Ruminant® (a blend of plant extracts), EnterixTM (garlic and citrus extracts), SilvAir™ (a nitrate-based additive), and red seaweed extracts produced by CH4 Global, Symbrosia, FutureFeed, Dulabio, and Volta Greentech.
The most significant development and market capture is DSM’s 3-NOP or Bovaer®. First approved for commercial use by Brazilian and Chilean authorities in 2021, it received EU market approval for dairy cows in 2022, and as of June 2024 is approved and available in 65 countries, including the recent additions of the USA and Canada. Dsm-firmenich is rapidly scaling up production capacity for Bovaer®, with a major new production facility underway in Scotland. Global commitments to reducing emissions have contributed to the relatively fast-track development of such solutions and the swift uptake of such products.
The methane reduction potential of 3-NOP
Life-cycle assessments over eight years or so have confirmed the sustained inhibitory effect of 3-NOP on methane production (Alemu et al. 2021). Applied in small doses (60 to 200 mg/kg of dry matter intake [DMI]), on average, 3-NOP decreases methane production in beef and dairy by 30 percent ((Dijkstra et al. 2018; Kim et al. 2020), although decreases of 80 percent or greater have been obtained in some studies with high-concentrate diets (Yu et al. 2021). The effect of 3-NOP on methane production is related to its level of inclusion in the diet (Yu et al. 2021) and diet composition (Kebreab et al. 2023; Dijkstra et al. 2018; Yu et al. 2021; Hristov et al. 2023). Some studies have observed a slight decline in effectiveness over time (Hristov et al. 2023). No residues occur in animal products (Hegarty et al. 2021) and no effects have been observed on manure emissions (Beauchemin et al. 2021). A one-year trial has confirmed the long-term effectiveness of Bovaer® (Gastelen et al. 2024), while further long-term studies are ongoing to address the concern of a potential reduction in the efficacy of methane inhibitors as rumen microbes adapt to supplementation over the long term (Expert Panel on Livestock Methane, 2024).Studies show thatdiet can have an impact on this efficacy (Gastelen et al. 2024). A partial life-cycle assessment of 3-NOP use on a dairy herd in California found a 13.7 percent reduction in GHG footprint of the herd (Feng and Kebreab 2020). Further studies in Australia and Canada state the carbon footprint of emissions associated with 3-NOP production can be up to 23 percent less than without these methane inhibitors (Hegarty et al. 2021). The use of 3-NOP, as with other methane inhibitors, can be easily combined with other mitigation strategies, providing opportunities to raise livestock productivity simultaneously.
6.1.1 Barriers to uptake of methane inhibitors
6.1.1.1 Insufficient investment and global cross-sector collaboration in product development
Product development has mainly relied on the private sector to cover costs, and it will likely take more than five years before products are broadly adopted if left to market forces. Insufficient production capacity is also a risk, even if new factories are under development. Though farmers are willing to test the use of methane inhibitors, few are reporting on GHG emissions reductions. Science-based protocols such as MiLCA (developed by the dairy sector to include the use of methane inhibitors and other mitigation technologies into life-cycle assessments) can encourage such reporting.
Additionally, there is limited technical know-how on using methane inhibitors and their impact, and there is a disconnect between commercial sector development of products and the farmers who should be using them. Briefs produced by organizations such as the Global Research Alliance (GRA), e.g., on feed additives, help to raise awareness. Still, national producer bodies in high-income countries are increasingly asking for more information on methane inhibitors, including how they work, animal and food safety, and optimal feeding regimes (e.g., UK NFU 2023). In low- to middle-income countries (LMICs), there is increasing interest but a lack of access due to high costs (Gomaa and Gado 2021; Maze et al. 2024).
6.1.1.2 Higher production costs when using methane inhibitors reduce incentives for adoption
The cost of methane inhibitors can be restrictive and limits their use to more high-value intensive beef production. Bovaer® has an additional cost of US$0.30 per head per day, and as there is little to no significant impact on the growth of animal or milk production, it increases the cost per unit of animal product, estimated around 5 percent extra feed cost for a dairy cow per day (Newbold et al. 2022).
Enteric methane reduction technologies are largely developed through collaborations between the private sector, including dairy producer companies and users, e.g., Danone, supported by GMH and Nestle. There are some emerging schemes, e.g., the US Department of Agriculture awarding US$89 million in funding to support farms using technologies like Bovaer® as incentives. However, there is ambiguity in carbon accounting rules that allow baselines to be moved to capture carbon payment advantages, reducing trust in carbon accounting processes. Feed additives are not yet included in the agriculture criteria of Climate Bonds Initiative’s and most national taxonomies, which would make them eligible for green finance. A feed additive calculator tool (FACT) considers carbon prices, costs, and animal performance impacts to advise farmers on the use of feed additives. Feed additive inclusion in carbon offsetting protocols should continue to be explored, as should feed additive – and more specifically methane inhibitor – deployment subsidies.
6.1.1.3 Insufficient information on meat and dairy consumers’ willingness to pay
There is limited information about consumers’ willingness to pay extra for products that directly reduce livestock methane emissions, including those that have used methane inhibitors. Those who are willing to pay extra for sustainable beef or other livestock may not be so willing to pay for meat produced using methane inhibitors. Resistance to change, concern about food safety aspects, or skepticism about the effectiveness of mitigation strategies such as feed additives can impede the uptake of technologies (Katare et al. 2023). Increasing consumer willingness to pay for such products requires investment both in terms of research and in raising awareness, as well as labeling. Meanwhile, some retailers are working to reduce the emissions intensity of their product range, including by working with suppliers to shift to using feed additives, but consumer-level awareness remains limited.
6.1.1.4 Current inappropriateness for extensive pasture-based systems
Methane inhibitors, including the current launch form of 3-NOP (Bovaer®), are most suited to livestock systems reliant on total mixed ration/feedlot and partial mixed ration plus partial grazing (6–8 hours per day) where exact feed control is possible. This has led to skepticism on the global potential of methane inhibitors by such as the FAO (FAO 2023a) because of the limited transferability to LMICs where extensive grazing systems are the norm. It is estimated that >50 percent of livestock intake is supplied by grazing (Wolf et al. 2021) and 9 percent of the world’s beef comes from grazing systems (de Haan et al. 1997).
Research is underway to develop slow-release methane inhibitors, including by DSM, working with companies such as Marks and Spencer UK. Recently, the Government of Ireland announced €1.4 million funding to progress government and academia collaboration on methane abatement in grazing systems. This includes trials on slow-release methane inhibitors called RumenGlas in beef and dairy cattle.
6.1.1.5 Limited collaboration on regulatory frameworks, sharing of data and metrics, reporting, and verification (MRV)
National regulatory frameworks governing the use of environmental (including methane) inhibitors is varied and ranges from none or very little regulation, to strict regulatory requirements. Regulation often depends on how these substances are used and integrated into feeding regimes (FAO 2023). At present there is no food safety maximum residue limit established by the Codex Alimentarius Commission to facilitate trade in products produced using the substances. New Zealand is promoting awareness raising within the Codex on environmental inhibitors and has convened two side events at relevant Codex committees to discuss the issues.
There has been an increase in standards and certifications by the private sector, such as from Cargill, causing confusion among producers and other value chain actors, and risking the credibility of a sector already under pressure. Some governments are also developing certifications; for example, Argentinian research institutes are working with the private sector and the International Environmental Product Declaration System to develop a national certification scheme (Winters 2024).
Delays in approvals can also be inhibiting. In the US, new drug approval processes can take 5 to 10 years and cost an average of US$30 million (EDF 2022). The EU provides an expedited regulatory approval process for products with a significant positive environmental benefit. Still, unless products are approved within the next two years and marketed immediately, they are unlikely to contribute to 2030 targets, emphasizing the need for an expedited process. Global regulatory frameworks that could speed up the approval processes and provide greater consistency across countries, together with more transparency and opportunities for monitoring, such as user payments, are needed. Within these regulatory frameworks, necessary standards and certifications can be developed.
Additionally, various data gaps hinder understanding of methane inhibitors’ progress and impact (Climate Policy Initiative 2023).2 Tracking investments in methane abatement has been taken up by the Climate Policy Initiative (Landscape of Methane Abatement Finance) but is not split by technology use, and projects are in the early stages. Tools such as GLEAM cannot quantify the impact of mitigation options. An improved GLEAM-X will offer various on-demand simulations under different scenarios while increasing access to more users. Data needs to be constantly updated, and farmers want farm-specific data to guide and monitor their production decisions. Information on feed, including embedded emissions numbers and feeding regimes, is limited. Global databases such as the Global Feed LCA database are consolidating some of this information. However, not all companies are willing to share necessary information. Developments such as a methodology for quantifying the reduction of methane emissions, including the application of feed supplements by Global Standard for the Global Goals, are in progress.
There is also a need to track technology uptake, with greater opportunity for tracking potential emissions reductions. Consistency of methodology for mitigation technologies is important for inclusion in countries’ national inventories. Most LMICs have significant data gaps across the board.
6.2 Reducing enteric methane emissions via low-methane forages
Improved forages, including legumes and grasses enriched with anti-methanogenic compounds (AMC) such as tannins, saponins, or flavonoids, offer a potentially cost-effective solution for reducing methane production from livestock systems, both pastoral and cut-and-carry (Bratta 2015), while meeting the increasing demand for livestock-based products (FAO 2018). These compounds can reduce methane emissions by inhibiting methanogenic microbes in the rumen and altering the microbial community to favor alternative fermentation pathways that produce less methane without negatively affecting animal productivity (Molina-Botero et al. 2024; Arndt et al. 2022).
Therefore, access to high-yielding, tropically adapted forages with methane mitigation potential can improve animal nutrition in grassland systems, leading to increased productivity and significantly lower total GHG emissions and emission intensities (emissions per unit of product) as well as increased incomes for farmers and pastoralists in developing nations (Arango et al. 2022; Costa Jr et al. 2022; Leyte et al. 2021; Seketeme et al. 2022; Paul et al. 2020).
Improved forages are already a part, though limited, of livestock systems in the global South (Fuglie et al. 2021). Adoption is particularly high in Latin America, with Brazil leading the way (Fuglie et al. 2021), and significant productivity benefits have been observed in areas that have adopted improved forages. Due to their adaptability to diverse agroecological conditions, the adoption and scaling of low-methane forage options could be facilitated. In an optimistic scenario, large-scale adoption of low-methane forages would reduce dairy animal methane emissions by 35 percent (Arndt et al. 2022).
In addition to reducing enteric methane emissions, these innovations could have several other environmental benefits, including improved carbon sequestration in soil and enhanced soil health with the potential for developing land-based GHG removal projects (Costa Jr et al. 2022; Paul et al. 2020).
Ongoing research and development (R&D) efforts through international cooperation on forage-based livestock production aim to identify and develop high-yielding, nutritious, and drought-tolerant forages to improve animal productivity and decrease enteric methane emissions through enriched AMC. These R&D efforts are focusing on three key areas:
- Identifying and promoting low-methane forage legumes with high levels of AMC directly into ruminant production systems in the global South.
- Breeding a methane emission-reducing trait into widely used forage grass cultivars and major cereals with feed value from silage and crop residues, either through conventional or precision breeding (gene-editing) approaches.
- Enabling deployment of low-methane forages into forage-based livestock (ruminant) systems in the global South.
International and national forage genebanks conserve seeds representing very high biodiversity. Most of the forage germplasm accessions conserved in these genebanks are legumes, and the low-methane legumes identified from these genebanks are ready for immediate use. The discovery of AMCs in low-methane legumes can pave the way for increasing AMC content in widely sown forage grasses through breeding or gene editing. International cooperation through the Global Methane Hub, including funding from the Bill & Melinda Gates Foundation and the Bezos Earth Fund, has enabled new investments in this area.
These grasses and fodder crops could, therefore, offer the potential for additional farmer income and increased milk availability while protecting the environment and posing no environmental risks (Fuglie et al. 2021). For example, smallholder dairy farmers in Africa will benefit from new forage varieties of nutritious forage grasses that increase productivity, improve soil health, and sequester carbon in deep soil layers.
Many countries are including food and feed management as a priority issue in their Nationally Determined Contributions (NDCs). There is a need to raise awareness of the potential of this low-methane forage technology.
6.2.1 Barriers to uptake of low-methane forages
Major barriers to the widespread adoption of improved forage systems, which would also need to be addressed with low-methane forages, include upfront costs when adopting new technology. These costs include: (i) working capital for planting improved forages and purchasing animals; (ii) capital investments in infrastructure, such as corrals, barns, and fencing; and (iii) opportunity costs of land and labor, which could limit adoption by some resource-poor farmers (Micol and Costa Jr 2023; White et al. 2013).
Farmers may also require training to effectively manage these new systems. Resistance from older farmers, who may be less open to changing traditional practices, further complicates the situation but could be addressed through farmer participatory research. Effective implementation requires supportive public policies and agreements between governments, which are often lacking (Micol and Costa Jr 2023; White et al. 2013).
Climate finance (e.g., grants, credit lines, impact funds, and carbon markets) can support de-risking investments and incentivize farmers (World Bank, 2021). Demonstrating and testing livestock productivity gains is essential to convince stakeholders of the benefits of adopting low-methane forages. Finally, solutions need to be tailored to specific regions – e.g., for adaptation to local soil and weather conditions – as a one-size-fits-all approach will not be effective.
6.3 Reducing emissions from fertilizer production via green ammonia
Global ammonia production in 2023 was estimated at around 183.6 million tons (IFA 2024). Ammonia manufacturing is estimated to contribute around 2 percent of global GHG emissions, emitting more than 2.4 tons of CO2 per metric ton of ammonia produced, four times as much as per ton of cement production. Ammonia is a key building block of many nitrogenous fertilizers. Around 85 percent of all ammonia is used to produce synthetic fertilizers (IRENA Innovation Outlook Renewable Ammonia 2022) but ammonia also has many uses in the petrochemical industry, i.e., plastic, paper, pharmaceuticals, and refrigerants, and the scope for mitigation thus goes beyond the agrifood sector.
The Haber–Bosch process, invented in the early 1900s, remains the primary source of ammonia from hydrogen and nitrogen. The process is highly energy intensive: it relies on hydrocarbons as a feedstock for producing hydrogen through steam methane reforming (or coal gasification), as well as for subsequently powering the energy-intensive ammonia synthesis process, which fixes nitrogen from the air with the hydrogen obtained from steam methane reforming (called brown ammonia).
Some improvements in the energy efficiency of ammonia production have occurred over time and can continue to occur, with newer plants and improved catalysts coming online. Phasing out ammonia production from coal (still widely used in some countries, with China being the main user) and relying only on natural gas would also reduce emissions. However, the process remains highly energy intensive. Carbon capture, utilization, and storage (CCUS) in fossil fuel-based ammonia production can lead to much more significant emissions reductions, with the Rocky Mountain Institute indicating that emissions can be reduced by more than 90 percent. So-called “blue ammonia” production combines large-scale ammonia production with CCUS and has been demonstrated to work worldwide. It is used or planned to be used by some of the major international fertilizer producers.
Zero carbon ammonia, called “green ammonia,” uses renewable energy to power electrolysis to produce hydrogen from water (replacing the steam methane reforming process based on hydrocarbon feedstocks) and the subsequent ammonia synthesis. Figure 5 depicts the difference in production processes of three different types of ammonia. Numerous green ammonia pilot plants have been set up, and the first large-scale green ammonia facilities may come online in the next five years. Startup companies have developed smaller, modular green nitrogen fertilizer plants, which are vastly less expensive than large plants and could facilitate decentralized nitrogen fertilizer production.
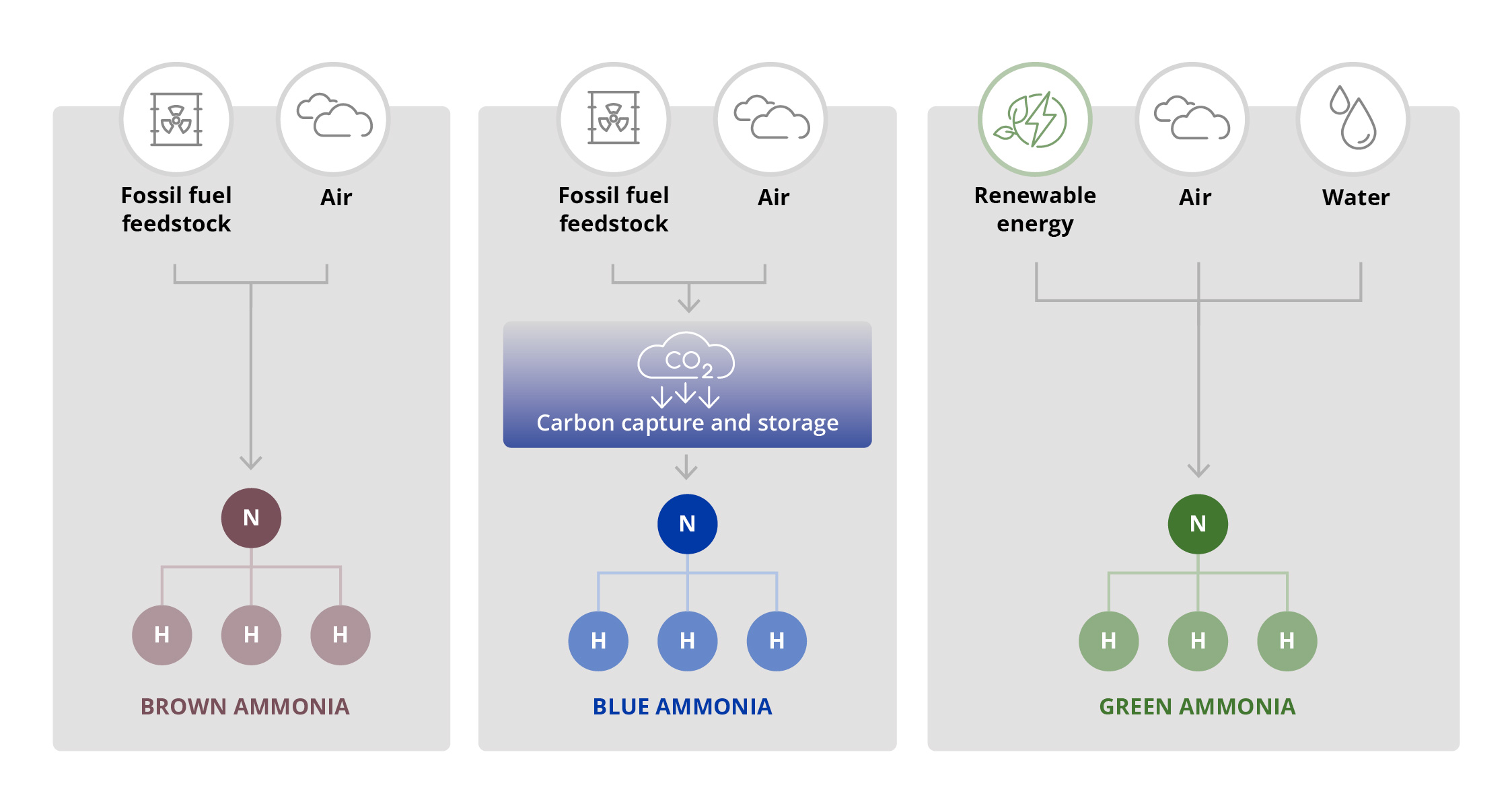
There are multiple green ammonia pilot plants and some larger-scale commercial plants now up and running. Between 2024 and 2028, the International Fertilizer Association (IFA) are tracking 55.9 Mt of ammonia capacity, of which 8.9 Mt is expected to be commissioned using electrolysis as feedstock (that is, green ammonia). According to their forecasts, the volume of projects under development but not yet expected to commission in the next five years is much larger, including 146 Mt of green ammonia capacity that could be commissioned after 2028 (IFA Short-Term Fertilizer Outlook 2023–2024).
Green ammonia’s commercial viability today is linked to the price of renewable energy, the capital cost of electrolysis, and government incentives. In some regions, the pricing point can be competitive, but the continued technological improvements in this space, coupled with the falling costs of electrolyzers and solar and wind power, will drive toward more general price competitiveness (Way 2022). Similarly, if the price of hydrocarbons continues to increase relative to renewable energy, the point of relative parity can be reached significantly faster (see Figure 6). Decentralized green ammonia production using affordable modular equipment can already be financially viable in geographies with low-cost renewable resource availability, due to savings from foregone transportation costs. A recent study found that the cost-competitiveness from green ammonia production is further enhanced by minimizing supply chain disruptions and estimates that it could become cost-competitive for up to 96 percent of global ammonia demand by 2030.
Further,green ammonia can be produced anywhere in the world with access to renewable energy. Today, most of the world’s ammonia is produced in countries with access to affordable natural gas or coal, resulting in highly centralized production centers. Fertilizer prices are tied to the cost of natural gas, and gas and fertilizer supply shocks reverberate worldwide, as last witnessed in the aftermath of the 2022 invasion of Ukraine. More ammonia plants using renewable energy would make the sector less prone to shocks.
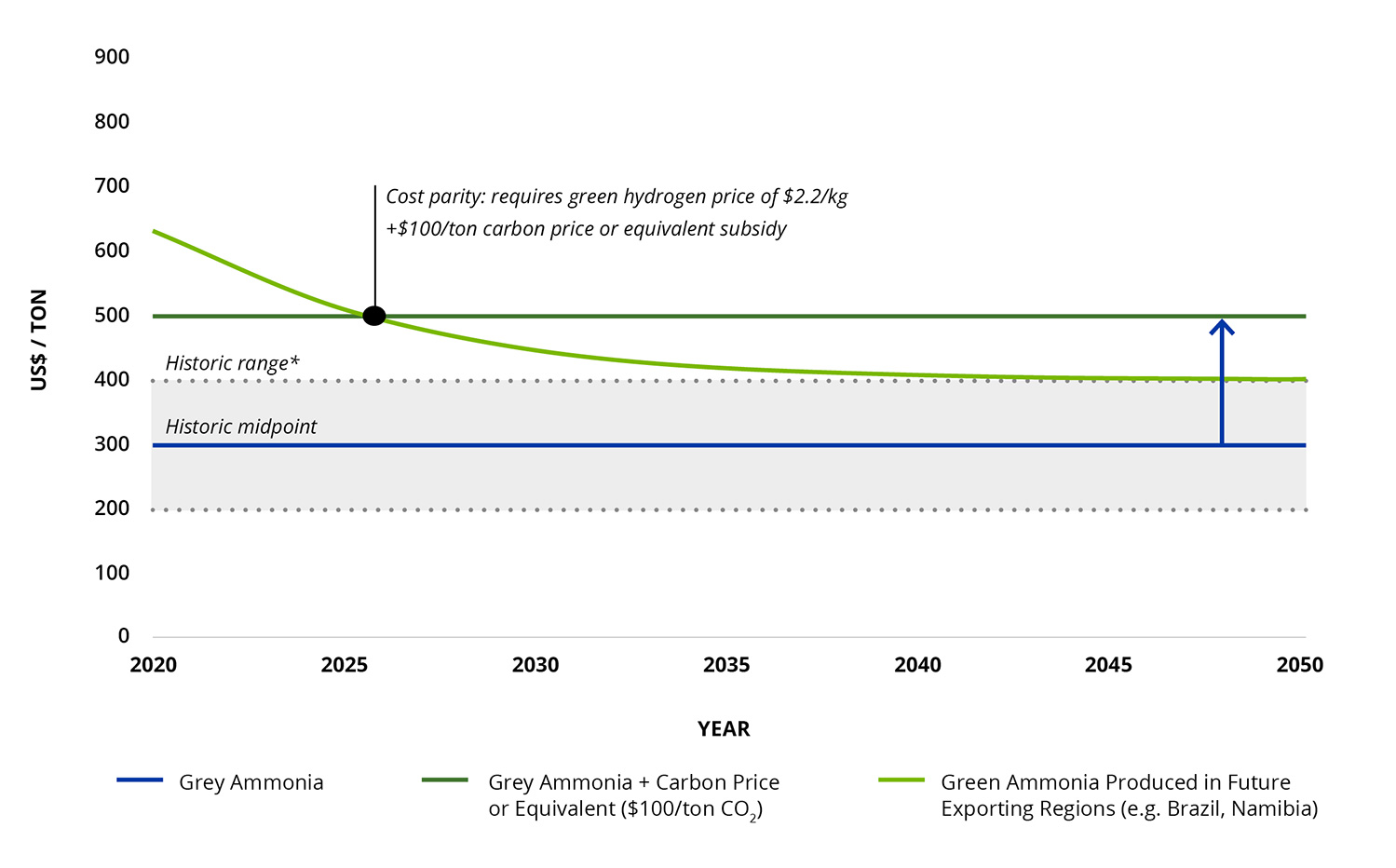
Smaller-scale green ammonia could be particularly relevant in developing countries and areas where transport and storage are costly or complex, particularly landlocked countries. Moreover, smaller plants can more easily be financed than large-scale ammonia plants.
Relatedly, green ammonia will also help to decarbonize the shipping industry, which could potentially result in an increase of up to five times in the world ammonia market. It could lead to a tighter global ammonia market, potentially affecting the nitrogen fertilizer supply of smallholder farmers. Development of small-scale decentralized nitrogen production is an option to minimize this risk.
6.3.1 Barriers to scaling of green ammonia
Considering that green ammonia today only makes up a tiny percentage of overall ammonia produced, it requires a hard look at possible barriers to adoption.
The cost of producing green ammonia is still higher than for blue ammonia (especially in the US, where blue ammonia is heavily subsidized by the Inflation Reduction Act), although over time, with an ongoing decrease in the price of renewable energy and electrolyzers, this barrier could be overcome. Given that between 10 and15 percent of global ammonia production is traded, countries may be hesitant to impose regulations mandating emissions reductions so as not to disadvantage their producers (noting that the EU only recently announced a plan to gradually phase out free emission allowances provided to EU fertilizer producers, but at the same time introducing carbon border tax adjustments).
The fact that blue ammonia (traditional ammonia production with CCUS) has gained significant traction is unsurprising since CCUS is a feasible option for large-scale, existing fertilizer producers with access to carbon dioxide sequestration infrastructure and, importantly, often also government financial support. Considering that fertilizer plants can operate for more than 40 years, operators of existing plants may not feel incentivized to switch to green ammonia production, preferring instead to pursue blue ammonia because it does not require any changes to their existing plants and operations, except for securing access to carbon dioxide sequestration infrastructure. The established large-scale ammonia producers may therefore find blue ammonia a preferred approach to lowering their carbon footprint: a large push on blue ammonia could make investments in green ammonia harder to come by, and relatively smaller green ammonia producers may find it difficult to compete with large industrial players using CCUS. On the other hand, larger industry players may find it advantageous to invest in or construct new green ammonia plants so as to further lower their remaining carbon footprint from producing blue ammonia. In addition, if green ammonia becomes the preferred fuel of the shipping industry (a quite likely scenario beyond 2030), the new capacity needed will all be green ammonia. Some countries are also investing in green ammonia to substitute imports: for example, Morocco, to substitute their large ammonia imports to produce ammonium phosphates as well as fertilizers containing nitrogen, phosphorus, and sulfur (NPS) and nitrogen, phosphorus, and potassium (NPK).
There are also important safety and security considerations in the ammonia production process, storage, and transport, as well as at the level of on-farm application of fertilizers. Anhydrous ammonia, the end product of green ammonia plants, requires careful handling and specialized equipment for transportation and application at the farm level for safety reasons. Today, it is widely used mainly in the United States and parts of Canada and Brazil. Ammonia codes of practice and government regulations can mitigate against such risks, as can proper equipment, but this implies additional costs for value chain players, including farmers, and smallholder producers in particular. In fact, for safety reasons, direct use of anhydrous ammonia by smallholders is not an option. Safety concerns can also be mitigated by converting anhydrous ammonia into aqueous ammonia (ammonia water solution), which is not an overly complex process, but transporting aqueous ammonia is not cost-effective. Converting ammonia into stable products such as urea, ammonium nitrate, ammonium sulfate, or ammonium phosphate is another well-known strategy to facilitate the transport and use of nitrogenous fertilizers.
Urea remains the most widely used fertilizer worldwide. While urea is a derivative of ammonia, an external source of carbon dioxide is required to produce urea from ammonia (in traditional ammonia production, carbon dioxide is a byproduct of the steam reforming process, which can then be used in producing urea). Urea cannot be produced with green ammonia as there is no carbon dioxide byproduct, meaning the preferred nitrogen fertilizer used by farmers globally might no longer be available if there is a shift to green ammonia.
It is worth mentioning here that combining production of green nitrate using plasma technology to develop green ammonium nitrate holds the potential to be a zero carbon or close to zero carbon nitrogenous fertilizer.
Balanced fertilization is important for agricultural productivity, soil health, and sustainability, and further processing would be required to produce multinutrient fertilizers (nitrogen, phosphorous, potassium, and other micronutrients). These considerations imply that setting up green ammonia plants alone is not sufficient, and additional processes and costs would be required to provide fertilizer products that farmers prefer and that provide for more balanced plant nutrition.
6.4 Reducing emissions from fertilizer application via site-specific nutrient management (SSNM)
Fertilizer application on the field is responsible for two-thirds of the emissions from fertilizers, while fertilizer production contributes to one-third of the emissions. Site-specific nutrient management (SSNM) is an approach to achieve increased NUE through the precise application of balanced fertilizer inputs, leading to higher crop productivity and other environmental benefits (Dobermann and White 1998; Dobermann et al. 2002), and thereby to reduce emissions from field application. SSNM is an important axis of integrated soil fertility management (ISFM). ISFM aims at maximizing agronomic use efficiency of the applied nutrients and improving crop productivity, and is built on two pillars: (i) a set of generic soil fertility management practices that necessarily include the use of fertilizer, organic inputs, improved germplasm, good agronomic practices, and other amendments such as lime and (ii) combining these practices with the knowledge on how to adapt them to local conditions (Vanlauwe et al. 2010).
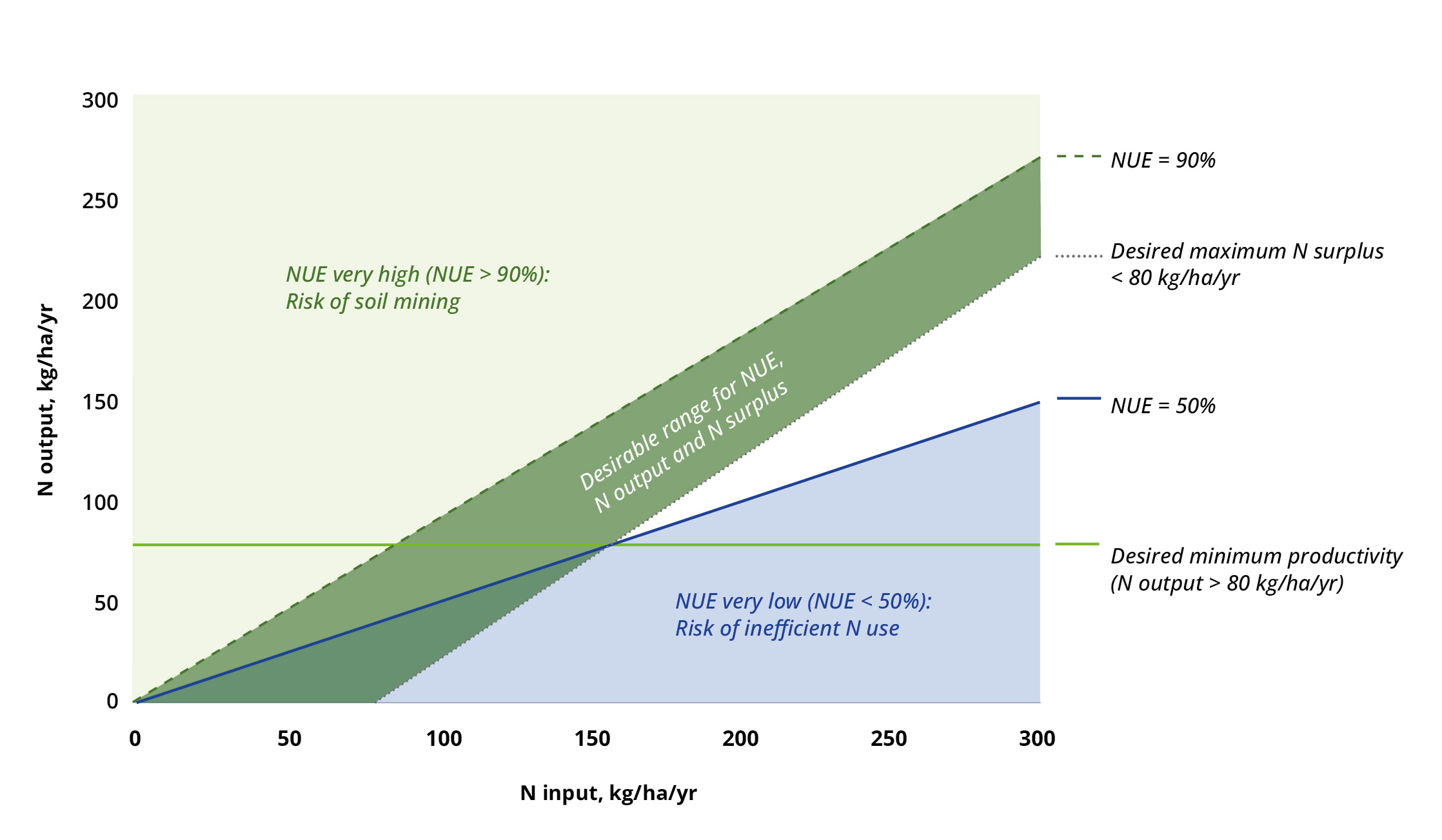
A recent review (Dreyfus et al. 2023) based on published literature has provided compelling evidence on the technical maturity, financial viability, and broad applicability of SSNM across geographies. Further, in a meta-analysis covering 11 countries in Africa and Asia, Chivenge et al. (2021) demonstrated that SSNM increased yields by 12 percent for maize, rice, and wheat and led to a reduction in nitrogen application by 10 percent and an increase in profitability of 15 percent at farm level.
Supported by advances in big data science, crop modeling, and geospatial analytics, and with the increasing availability of remote sensing products, SSNM is guided by an array of decision support tools that allows guidance on the right source at the correct rate, right time, and in the right place, i.e., the 4Rs (Johnston and Bruulsema 2014) to achieve site-specific nutrient management, which includes maximizing the use efficiency of nitrogen. The right fertilizer time means applying the fertilizer at the right stage of crop growth for improved uptake. It may also entail split application during the season to avoid losses. The right fertilizer place entails applying the fertilizer in the right location considering, for example, topography and the crop’s growth habit and also foliar versus soil application. In some cases, incorporation of fertilizer in the soil is recommended to avoid losses. Efficiently used fertilizer will ensure that available nitrogen in the soil is minimal, thus reducing the potential for nitrous oxide emissions (Tian et al. 2020).
GHG emissions reductions are also observed with the implementation of SSNM. For instance, SSNM recommendations reduced nitrogen fertilizer application by 40 percent and reduced nitrous oxide emissions by 66.8 percent in cotton production in India (Gupta et al. 2022). Similarly, Pampolino et al. (2007) reported that reductions in fertilizer use with SSNM averaged 10 percent in the Philippines and 14 percent in Vietnam. Additional evidence from on-farm trials in India showed higher yields and lower total estimated GHG emissions per ha under SSNM compared with farmer practice (Sapkota et al. 2014; Zhang et al. 2018). Nitrogen application rate was reduced by 24 percent and nitrous oxide emissions by 19 percent under maize production in China over a nine-year experiment (Huang et al. 2021). In the Indo-Gangetic Plains, current evidence suggests using Nutrient Expert® (see below) reduces the nitrogen application rate by 18 percent with SSNM (Sapkota et al. 2021). However, when SSNM tools recommend increased fertilizer input due to low baseline conditions (as in much of Africa), an increase in GHG emissions will ensue (Leitner et al. 2020), and is acceptable given the very low historical emissions from the continent, where improving food security is a higher priority (Falconnier et al. 2023). That said, the land-sparing effect of closing yield gaps can counteract the effect of the increased emissions from agricultural inputs; Africa is the region that witnessed the largest cropland expansion between 2003 and 2019 and is also projected to have the largest agricultural expansion to 2050 (Potapov et al. 2022).
Various decision support tools exist to support SSNM. Some examples include:
- Nutrient Expert®, which is an interactive, computer-based decision support tool that estimates the attainable yield for a farmer’s field based on the growing conditions, determines the nutrient balance in the cropping system based on yield and fertilizer/manure applied in the previous crop, and combines such information with expected nitrogen, phosphorus, and potassium response in target fields to generate location-specific nutrient recommendations.
- Crop Manager is a computer- and mobile phone-based application that provides small-scale rice, rice-wheat, and maize farmers with site- and season-specific recommendations for fertilizer applications focused on Asia.
- RiceAdvice is an Android-based decision support tool that provides farmers with pre-season field-specific management guidelines for African rice production systems. The tool has guidelines on target yield, nutrient management, crop calendar, and good agricultural practices.
- AKILIMO provides evidence-based agronomy solutions at scale, addressing major agronomic decisions, applying state-of-the-art data analytics, implementing advanced statistics, mechanistic and empirical methods, and optimizing for profitability for cassava.
- AgWise is a framework that applies process-based crop models, machine learning algorithms, cutting-edge statistical models, and remote sensing technologies to address challenges enhancing food security, climate resilience, and sustainable resource use to improve soil health. AgWise is being integrated into different partner solutions through application programming interfaces (APIs).
- The Environmental Defense Fund (EDF) N Balance model: The model helps to calculate the N balance (N added minus N removed) and shows the amount of N left over and at risk of being lost to the environment. The model can be applied broadly across all crops in temperate climates, regardless of soil type or N source, including synthetic and organic N fertilizer.
6.4.1 Barriers to adoption of SSNM
SSNM decision support tools are heavily dependent on data availability. The lack of standardized and open data hinders the application of big data science to decision support tools with sufficient accuracy/resolution, especially in low- and middle-income countries. The accuracy of these tools needs to be further improved to minimize the risk of under- or non-performance, as the performance of these tools is strongly related to data quality and availability.
There is limited bundling of decision support tools with other agro-inputs (e.g., seeds, mechanization), limiting the achievement of agronomic gains in terms of yields and other key performance indicators, which in turn makes it harder for farmers to adopt these tools.
The adoption of SSNM is closely tied to the availability of advanced agricultural technologies including digital decision support tools. The cost of these, coupled with limited digital literacy, can be prohibitive for many smallholder farmers.
The lack of sustainable business models, including revenue generation options, limits the sustainable deployment of decision support tools at scale. There are limited financing models to fund the hosting data and tools. Notably, in LMICs, the cost and accessibility of fertilizer are major issues, and strong input markets are a prerequisite for SSNM, along with the availability of local nutrient sources such as manure and compost.
There are inadequate policies and incentives to encourage and facilitate the development of digital tools to support SSNM. Government support and subsidies can play a crucial role in promoting these practices.
The initial investment in SSNM decision tools such as GPS-guided machinery, plus fluctuating crop and fertilizer market prices, reduces the cost-effectiveness and incentives for adoption.
1 It is also important to note that, despite increased agricultural productivity over the last several decades, hunger and malnutrition in all forms have continued to rise. Cropland expansion has primarily not been for direct food consumption but for biofuel and livestock feed. In addition, a third of all food produced is lost or wasted. Addressing these three trends will do more to meet growing food demand than solely focusing on agricultural productivity.
2 For example, an inability to quantify and link methane reductions to tracked finance (particularly complex with livestock due to fragmented value chains), limitations in assessing intent during screening process, a lack of standardized reporting, difficulty distinguishing methane finance from usual expenditure, variations in reporting practices across sources and entities, and a lack of alignment in investment needs assessment.